As the world turns to solar energy as a clean, renewable power source, understanding the factors that influence solar panel performance becomes important. One of the most significant yet often misunderstood factors is temperature. In this guide, we’ll explore the relationship between solar panel efficiency and temperature, diving into the science, practical implications, and strategies for optimizing performance.
Solar Panel Efficiency
Solar panel efficiency refers to the amount of sunlight that a panel can convert into usable electricity. For example, if a solar panel has an efficiency rating of 20%, it means that 20% of the sunlight hitting the panel is converted into electrical energy, while the rest is reflected or lost as heat.
Most commercially available solar panels have efficiency ratings between 15% and 22%, with some high-end models reaching up to 25%. These ratings are typically measured under standard test conditions (STC), which include a temperature of 25°C (77°F), solar irradiance of 1000 W/m², and an air mass of 1.5. It’s important to note that these conditions rarely reflect real-world performance, especially when considering temperature variations.
The Physics Behind Solar Cell Efficiency
To understand the impact of temperature on solar panel efficiency, we need to look at the physics of how solar cells work. Solar cells operate based on the photovoltaic effect, a phenomenon where certain materials generate an electric current when exposed to light.
In a typical silicon solar cell, the absorption of photons creates electron-hole pairs. These charge carriers are then separated by the cell’s electric field, creating a flow of electrons through an external circuit. The efficiency of this process is determined by several factors:
- Bandgap Energy: This is the minimum energy required for an electron to break free from its bound state and participate in conduction. Silicon has a bandgap of about 1.1 eV, which is well-suited for capturing a broad range of the solar spectrum.
- Recombination: This occurs when electrons and holes recombine before they can be collected, reducing efficiency. Higher temperatures increase the rate of recombination.
- Series Resistance: This represents the resistance to current flow through the cell and external circuit. It increases with temperature, reducing efficiency.
- Shunt Resistance: This represents alternative pathways for current to flow, bypassing the intended circuit. Lower shunt resistance reduces efficiency.
Understanding these principles helps explain why temperature has such an impact on solar cell performance.
The Temperature Coefficient: A Key Metric
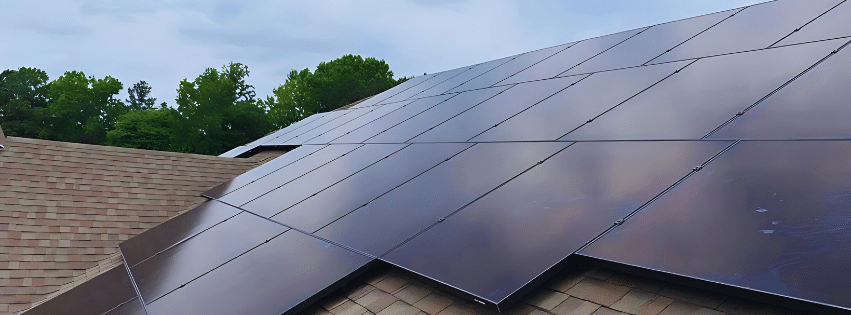
When discussing solar panel efficiency and temperature, one crucial term to understand is the “temperature coefficient.” This metric quantifies how much a panel’s power output changes for each degree Celsius change in temperature above or below 25°C.
The temperature coefficient is expressed as a percentage per degree Celsius. For example, a temperature coefficient of -0.5% per °C means that for every degree above 25°C, the panel’s power output decreases by 0.5%.
It’s important to note that there are different temperature coefficients for different panel characteristics:
- Power Temperature Coefficient: This is the most commonly cited, representing the change in overall power output.
- Voltage Temperature Coefficient: This represents the change in open-circuit voltage (Voc).
- Current Temperature Coefficient: This represents the change in short-circuit current (Isc).
Usually, the voltage coefficient is negative (voltage decreases with temperature), while the current coefficient is slightly positive. The overall power coefficient is negative, indicating decreased efficiency at higher temperatures.
Higher Temperatures, Lower Efficiency
Contrary to what one might expect, solar panels actually become less efficient as they get hotter. This inverse relationship between temperature and efficiency is due to the physics of how solar cells work.
As the temperature increases, the electrons in the solar cell become more energetic, reducing the bandgap of the semiconductor material. This leads to several effects:
- Decreased Open-Circuit Voltage: The most significant effect is a reduction in the cell’s open-circuit voltage. This decrease is typically around 2.2 mV per °C for silicon cells.
- Slightly Increased Short-Circuit Current: The current increases slightly due to increased carrier concentrations at higher temperatures. This small increase is outweighed by the voltage decrease.
- Increased Recombination: Higher temperatures lead to increased recombination rates, where electrons and holes recombine before they can be collected.
- Increased Resistance: The internal resistance of the cell increases with temperature, further reducing efficiency.
The combined result of these effects is a net decrease in power output and efficiency as temperature rises.
Quantifying the Impact
The extent of efficiency loss due to temperature varies depending on the specific type of solar panel and its temperature coefficient. To give a general idea:
- A typical crystalline silicon solar panel might lose 0.3% to 0.5% of its efficiency for every 1°C increase in temperature above 25°C.
- On a hot summer day where panel temperatures might reach 60°C (140°F), this could translate to a 10-15% decrease in power output compared to the panel’s rated efficiency.
- In very cold conditions, solar panels can actually perform above their rated efficiency. For example, at 0°C (32°F), a panel might produce 5-7% more power than its rated output.
It’s worth noting that while efficiency decreases with temperature, the total energy output might still be higher on a hot, sunny day compared to a cool, cloudy day, simply due to the increased solar irradiance.
Factors Influencing Panel Temperature
Several factors contribute to the operating temperature of a solar panel:
- Ambient Air Temperature: The surrounding air temperature is a primary factor. Panels will typically operate at 20°C to 40°C above the surrounding air temperature.
- Solar Irradiance: More intense sunlight leads to higher panel temperatures. Under full sun conditions, panel temperatures can easily reach 50-65°C.
- Wind Speed: Wind can help cool panels, potentially improving efficiency. Studies have shown that wind speeds of 1 m/s can reduce panel temperature by 5-11°C.
- Installation Method: Roof-mounted panels typically run hotter than ground-mounted or rack-mounted panels due to less air circulation. The difference can be 5-10°C.
- Panel Color and Material: Darker panels and certain materials absorb more heat. For example, black-framed panels might operate 1-3°C hotter than silver-framed panels.
- Panel Efficiency: Ironically, more efficient panels tend to run cooler as they convert more sunlight to electricity rather than heat. High-efficiency panels might operate 2-5°C cooler than standard panels under the same conditions.
- Time of Day: Panel temperatures typically peak in the early afternoon, lagging behind peak solar irradiance by 1-2 hours.
- Geographical Location: Latitude, altitude, and local climate conditions all play a role in determining panel operating temperatures.
Understanding these factors is crucial for managing solar panel performance in various environments.
Strategies for Reducing Temperature Effects
Understanding the impact of temperature on solar panel efficiency allows for the development of strategies to lessen these effects:
- Proper Ventilation: Making sure there’s adequate airflow around panels can help dissipate heat. This is one reason why many installations include a gap between the roof and the panels. Studies have shown that increasing the air gap from 2 cm to 20 cm can reduce panel temperature by up to 10°C.
- Light-Colored Roofing: For roof-mounted systems, using light-colored roofing materials can reduce overall heat absorption. A white roof can be 30°C cooler than a black roof on a sunny day.
- Temperature-Resistant Solar Panels: Some manufacturers produce panels designed to perform better in high-temperature conditions, with lower temperature coefficients.
- Floating Solar Farms: Installing solar panels on bodies of water can help keep them cool through evaporation and conduction. Floating solar installations have shown to be up to 11% more efficient than land-based systems in some cases.
- Hybrid PV-Thermal Systems: These systems capture the heat from solar panels and use it for water heating, simultaneously cooling the panels and providing an additional energy benefit. They can increase overall energy efficiency by 10-15% compared to separate PV and thermal systems.
- Time-of-Day Considerations: In very hot climates, solar panels might actually produce more total energy in the cooler morning and evening hours than at the hottest part of the day. This can inform system design and orientation.
- Active Cooling Systems: While less common due to cost and complexity, active cooling systems using water or forced air can significantly reduce panel temperatures. Some experimental systems have shown efficiency improvements of up to 15%.
- Phase Change Materials (PCMs): Incorporating PCMs into panel design can help absorb excess heat during peak temperatures. Research has shown that PCMs can reduce panel temperature by 5-10°C during peak hours.
- Anti-Reflective and Cooling Coatings: Specialized coatings can help panels reflect non-useful wavelengths of light, reducing heat absorption. Some coatings have been shown to reduce panel temperature by 5-10°C.
Implementing one or more of these strategies can help maintain higher efficiency levels even in challenging temperature conditions.
The Role of Inverters in Temperature Management
Inverters, which convert the DC electricity produced by solar panels into AC electricity for use in homes and the grid, also play a role in managing temperature effects. Modern inverters use maximum power point tracking (MPPT) technology to optimize the voltage and current from the solar array, helping to reduce some of the efficiency losses because of temperature.
Some advanced inverters even have temperature-compensated MPPT algorithms that adjust their operation based on panel temperature, optimizing performance across a range of conditions. These smart inverters can improve system efficiency by 1-3% compared to standard inverters.
Micro-inverters and power optimizers, which operate at the individual panel level, can help lessen the impact of temperature variations across an array. If some panels are hotter than others (due to shading or installation differences), these module-level power electronics can ensure each panel operates at its individual maximum power point.
Case Studies
To illustrate the real-world implications of temperature effects on solar panel efficiency, let’s consider a few case studies:
- Desert Solar Farm:
A large solar installation in the Mojave Desert faced significant efficiency losses during summer months when daytime temperatures regularly exceeded 40°C (104°F). Panel temperatures often reached 70°C (158°F). By implementing cooling techniques, including automated cleaning systems that also provided evaporative cooling, they were able to improve overall energy output by 5%. The cooling system reduced average panel temperatures by 15°C during peak hours.
- Alpine Solar System:
A solar array installed at a ski resort in the Alps actually overperformed its rated specifications during the cold, sunny winter months. With ambient temperatures often below freezing and panel temperatures around 10°C (50°F), the system regularly produced 10-15% more power than its rated capacity. The combination of high solar irradiance and low temperatures led to efficiency gains of up to 10% above rated values.
- Floating Solar in Japan:
A floating solar installation on a reservoir in Japan showed 11% higher efficiency compared to a similar land-based system nearby. The water-cooling effect kept panel temperatures an average of 10°C lower than the land-based system during summer months.
- Agrivoltaic System in France:
A combined solar and agricultural installation in France used raised solar panels to provide partial shade for crops. This not only improved crop yields but also kept the panels cooler. The panels in this system operated at temperatures 5-8°C lower than a conventional ground-mounted system, resulting in a 3% increase in annual energy production.
These cases highlight the importance of considering local climate conditions and implementing appropriate strategies when designing and implementing solar energy systems.
Future Developments
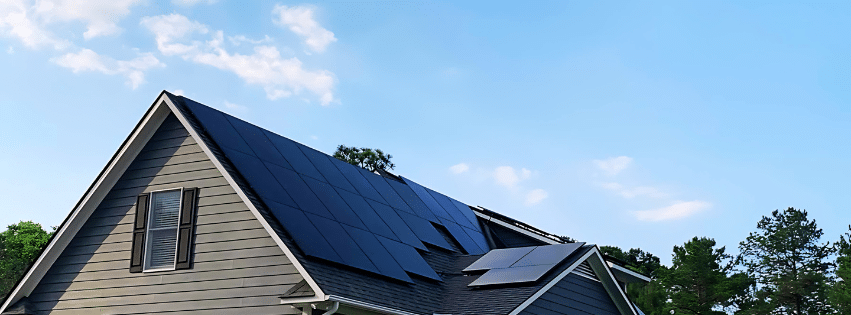
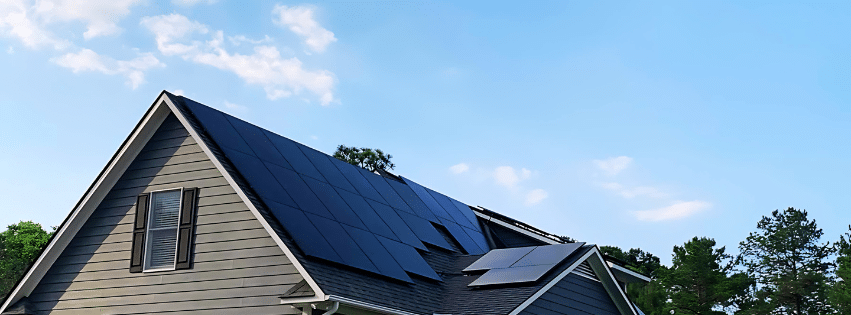
Research into improving solar panel performance at high temperatures is ongoing. Some promising developments include:
- New Materials: Researchers are exploring materials with better thermal properties for use in solar cells. For example, adding a few percent of guanidinium to the perovskite layer in solar cells has been shown to improve their heat resistance.
- Nanotechnology: Nanostructures that can help dissipate heat more effectively are being developed. For instance, carbon nanotube arrays on the back of solar cells have shown potential to improve heat dissipation.
- Thermophotovoltaic Cells: These advanced cells aim to capture energy from both light and heat, potentially turning high temperatures from a problem into an advantage. Some experimental designs have shown efficiency improvements at temperatures above 400°C.
- Smart Coatings: Self-cooling coatings that can reflect certain wavelengths of light when temperatures rise are in development. One such coating developed by Stanford University researchers could lower panel temperatures by up to 5°C.
- Artificial Intelligence: AI-driven systems that can predict and adapt to temperature fluctuations, optimizing panel orientation and cooling strategies in real-time. These systems have shown potential to improve overall system efficiency by 2-3%.
- Hybrid Systems: Combining solar PV with other technologies,like thermoelectric generators, to capture and utilize waste heat. Some experimental hybrid systems have shown overall efficiency improvements of up to 10%.
- Advanced Thermal Management: Innovations in heat sink design and active cooling technologies specifically for solar panels. For example, microfluidic cooling channels integrated into the panel structure have shown promise in laboratory tests.
- Luminescent Solar Concentrators (LSCs): These devices can concentrate light without the heat build-up associated with traditional concentrators, potentially allowing for higher efficiency without temperature-related losses.
As these technologies mature, we can expect to see improvements in the temperature resilience of solar panels, leading to more efficient solar energy systems across a range of climatic conditions.
Navigating Challenges with 8MSolar
The relationship between solar panel efficiency and temperature is complex and multifaceted. While higher temperatures do lead to decreased efficiency, this challenge is not hopeless. Through careful system design, selection of appropriate technologies, and implementation of innovative cooling strategies, it’s possible to reduce much of the negative impact of high temperatures on solar panel performance.
This is where the expertise of companies like 8MSolar becomes imperative. With our understanding of solar technology and its real-world applications, 8MSolar is at the forefront of addressing the temperature-efficiency challenge in solar energy systems.
At 8MSolar, we recognize that each solar installation is unique, facing its own set of environmental and technical challenges. Our team of experienced engineers and solar experts approaches each project with a unique perspective, considering not just the panels themselves, but the entire ecosystem in which they operate. This includes:
- Advanced System Design: We utilize cutting-edge modeling software to predict temperature effects and design systems that optimize performance across all seasons and weather conditions.
- Technology Selection: Our partnerships with leading manufacturers allow us to select and offer solar panels and inverters with superior temperature coefficients and advanced cooling technologies.
- Innovative Cooling Solutions: From passive ventilation designs to more advanced active cooling systems, we implement strategies tailored to each installation’s specific needs and local climate conditions.
- Smart Monitoring Systems: We equip our installations with sophisticated monitoring tools that track performance in real-time, allowing for rapid response to any temperature-related efficiency drops.
- Continuous Research and Development: Our team stays at the forefront of solar technology, constantly evaluating and incorporating new advancements in temperature-resistant materials and cooling techniques.
The 8MSolar difference lies not just in our technical expertise, but in our commitment to sustainable energy solutions that stand the test of time and temperature. We understand that for solar energy to truly fulfil its promise as a cornerstone of our clean energy future, it must perform reliably and efficiently in all conditions.
As we look to the future, the challenge of maintaining solar panel efficiency in the face of rising global temperatures will only become more critical. At 8MSolar, we’re not just responding to this challenge – we’re anticipating it, innovating to meet it head-on, and turning potential obstacles into opportunities for advancement.
In embracing solar energy, we’re not just adopting a new technology – we’re cultivating a brighter, cleaner future for our planet. And with partners like 8MSolar leading the way in addressing challenges like temperature-related efficiency losses, that future looks brighter than ever.
Contact 8MSolar today to learn how we can help you harness the full power of the sun, come rain or shine, heat or cold. Together, we can build a more sustainable and energy-efficient world, one solar panel at a time.