Scientists have proposed a building-integrated PV system that integrates airflow to cool the panels and regulate room temperature. The system, which also acts as a shading device, can reportedly mitigate the decline in power generation efficiency without additional energy consumption.
Researchers from Japan’s Nagoya University have proposed a new building-integrated photovoltaic (BIPV) system that integrates an airflow-type PV integrated sun shading device (PVSD) with ventilated slats.
The system enables passive cooling and heat recovery without additional energy input. “An airflow type PVSD is a facade system designed with openings at the top and bottom of the louvers, allowing air to pass through.” the team explained. “During the cooling period, outside air was introduced at the bottom of the slats to facilitate the passive cooling of the solar panels. As the outside air rose through the louvers, it passively cooled the solar panels and then exited through the top of the louvers. During the heating period, the indoor air was introduced through the underside of the slats and heated via heat exchange with the solar panels. Heated air was blown into the room through the top of the slats.”
Image: Nagoya University, Applied Energy, CC BY 4.0
The prototype system consists of a 200mm x 1,500mm solar PV panel installed in front of seven aluminum sun protection slats. The solar panel, which uses monocrystalline cells, has a nominal power of 39 W and an efficiency of 13%. Opening holes allows air to flow into the system. A second prototype included all of the above features, but had no airflow openings.
Image: Nagoya University, Applied Energy, CC BY 4.0
The system’s performance was measured on a day in January 2022 in Shizuoka City.
“At 11:40 am, when solar radiation was at its peak, the non-airflow type PVSD generated 242.21 W, while the airflow type PVSD produced 247.25 W. This indicates that the electricity generation by the airflow type PVSD did not occur significantly. change. The slight improvement in electricity generation can be attributed to the low outdoor temperatures, which did not affect the increase in the surface temperature of the PV panels,” the team said. “However, it is expected that in actual buildings where multiple units are installed, the difference in energy output between airflow and non-airflow type PVSDs will be greater.”
After the actual measurements, the team performed numerical modeling via the EnergyPlus software. In the single-room model, seven PVSDs were installed in two window locations. Three study cases were tested, namely a system without PVSD, a system with PVSD without airflow and a system with PVSD with airflow. The software simulated a year of operation and found that measured solar panel temperatures and exhaust air temperatures were within approximately 20%.
In the case without PVSD, the annual cooling consumption was 386 kWh, the heating power 58 kWh and the lighting required 295 kWh, for a total consumption of 739 kWh. In the case of the PVSD without airflow, cooling, heating and lighting were 364 kWh, 65 kWh and 391 kWh respectively, while the PV generated 496 kWh for a total net consumption of 324 kWh. The airflow PVSD had the lowest net consumption of 303 kWh; that is, it produced 503 kWh and consumed 364 kWh in cooling, 50 kWh in heating and 391 kWh in lighting.
“Compared to the PVSD without airflow, installation of the PVSD with airflow increased PV generation by approximately 1.4% and reduced heating demand by approximately 29%,” the researchers pointed out. “The results of the annual calculations indicated an improvement in the efficiency of electricity generation and the effects of indoor heating with the air flow type PVSD.”
Image: Nagoya University, Applied Energy, CC BY 4.0
After proving that the airflow type PVSD produces the best results, the team focused on optimizing it for installation angles, heights and opening areas. The opening areas were set to 0.0017 m², 0.0051 m², 0.0068 m², 0.0085 m² or 0.0102 m², which are 0.5, 1.5, 2.0, 2.5 and 3, respectively. 0 times the original opening of 0.0034 m² in the prototype. The height was set to 0.8 m, 10 m, 20 m, 30 m, 40 m, 50 m or 60 m above ground level, and the installation angle was set to 70◦, 75◦, 80◦, 85◦ or 90 ◦ , relative to the ground.
“Multiplying the openings by 3.0 times resulted in the highest PV production, with an increase of 1.2 kWh/y compared to the values in the prototype model,” they said. “The demand for heating showed a logarithmic decrease with the increase of the opening area. A multiplication by 3.0 times resulted in the lowest heat demand, with a decrease of 4.6 kWh/y compared to the values in the prototype.”
Regarding the different heights, PV production reached its maximum at 60 m, with an increase of 14 kWh/y compared to the installation at 0.8 m above ground level. At this last height, the demand for heating was lowest and decreased by 7.7 kWh/y compared to the installation at 60 meters above the ground. The difference in PV production between the airflow-type PVSD and the PVSD without airflow increased as the inclination angle decreased, reaching a maximum difference of 10.5 kWh/y at 70◦.
The system was presented in “Development and verification of a photovoltaic integrated airflow type sun shading device on building facades”, published in Applied energy.
This content is copyrighted and may not be reused. If you would like to collaborate with us and reuse some of our content, please contact: editors@pv-magazine.com.
Popular content
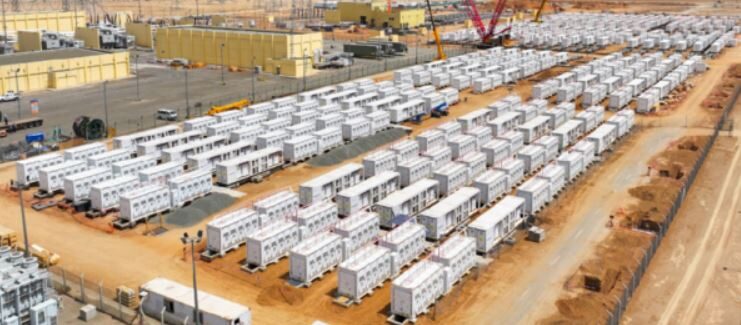